1.5 Hitching a Ride with the Molecules: The Mechanics of Smell
![]() |
The things we can smell are actually molecules with specific properties. Pictured above is a model of an ethyl phenylacetate molecule. This is an ester compound with a honey like odour. Esters are often aromatic, and are responsible for many of the aromas of fruits and herbs, as well as some of the aromas found in wines and spirits. |
The telephone, the motion picture and the computer are some of the better known technologies of the last two centuries that have been inspired by a fascination for how we see, hear and think. In the early 20th century, a less celebrated but equally remarkable machine was to anticipate our understanding of the chemical senses of taste and smell. Known as 'Gas Chromatographs', they allowed scientists to tell what chemical compounds were present in smog, cigarette smoke, petroleum or even the aroma of coffee. Amongst other significant discoveries, they gave chemists the ability to synthesize proteins such as insulin, and understand how plants use the sun's energy to make food. Physicists used them to chart the periodic table and develop atomic energy. In the 1940s, when commercially viable machines began to appear, their value in odour analysis was recognised. Inevitably they made their way into the perfume and wine industries.
Today we can better understand why a wine smells the way it does by feeding samples into a 'gas liquid chromatograph' with a set of chosen criteria to identify and quantify. As in the simpler process of distillation where small molecules fly out first, followed by the big ones (so alcohol is separated from water), the sample is heated to evaporation in order to release volatile compounds. Since all the molecules of a given structure will behave more or less identically, they exit the machine collectively and at the same time. As each 'puff' of molecules is released, a computer generated graph peaks corresponding to the most abundant chemical compounds. The higher the peak, the greater the number of molecules of a certain type are present.
Software associated with gas chromatographs can now chemically match 'similar' profiles within a few seconds. While their accuracy varies from machine to machine, the technology is reliable and getting better. It's even been suggested they threaten to undermine the role of wine critics. But like all machine based tests it has limitations. Humans can detect certain chemical compounds to an accuracy of as little as three parts per trillion, (think of the size of a finger nail over the diameter of the earth). For us, even minor differences between aromas are capable of significantly altering our sensations. ** Recognising this fact, some scientists have integrated the sensitivity of the human nose with the reliability of the machine. A proportion of the volatile molecules are trapped within a chamber with an outlet that allows a human 'sniffer' a means of comparing their opinions with the machine output to obtain a more 'rounded' result.
The mechanics of gas chromatography reflect modern day research that has revealed how our bodies work with the molecular compounds called 'odorants'. We know now that for an odour molecule to enter our bodies and be deemed 'odorous', it has to be volatile, meaning, it readily evaporates. Secondly, for an odour molecule to be absorbed by the body, it must have some water solubility and also some ability to be dissolved in fat. These properties all aid in the transferal of a scent molecule through our noses and 'into our brains'. If a molecule doesn't have these properties, or they're not sufficiently present, we simply can't smell it.
Scientists refer to the act of smelling as 'olfaction'. In the table below are some commonly occurring odorous compounds, their corresponding scent description and where they can be found in nature (if applicable):
Compound Name | Fragrance | Natural Occurrence |
Methyl Formate | Ethereal | |
Methyl Acetate | Sweet nail polish, solvent | |
Methyl Butyrate, Methyl Butanoate | Fruity, apple, pineapple | |
Ethyl Acetate | Sweet solvent | Wine |
Ethyl Butyrate, Ethyl Butanoate | Fruity, orange, pineapple | |
Isoamyl Acetate | Fruity, banana, pear | |
Pentyl Butyrate, Pentyl | Fruity, pear, apricot | |
Pentyl Pentanoate | Fruity, apple | |
Octyl Acetate | Fruity, orange | |
Myrcene | Woody, complex | Verbena, bayleaf |
Geraniol | Rose, flowery | Geranium, lemon |
Nerol | Sweet, rose, flowery | Neroli, lemongrass |
Citral Lemonal, Geranial, Neral | Lemon | Lemon myrtle, lemongrass |
Citronellal | Lemon | Lemongrass |
Citronellol | Lemon | Lemongrass, rose, pelargonium |
Linalool | Floral, sweet, woody, lavender | Coriander, sweet basil, lavender |
Nerolidol | Woody, fresh bark | Neroli, ginger, jasmine |
Limonene | Orange | Orange, lemon |
Camphor | Camphor | Camphor, laurel |
Terpineol | Lilac | Lilac, cajuput |
Alphalonone | Violet, woody | Violet |
Thujone | Minty | Cypress, lilac, juniper |
Benzaldehyde | Almond | |
Eugenol | Clove | Clove |
Cinnamaldehyde | Cinnamon | Cassia, cinnamon |
Ethyl Maltol | Cooked fruit, caramelised sugar | |
Vanillin | Vanilla | Vanilla |
Anisole | Anise | Anise |
Anethole | Anise | Anise, sweet basil |
Estragole | Tarragon | Tarragon |
Thymol | Thyme | Thyme |
Trimethylamine | Fishy, ammonia | |
Putrescine Diaminobutane | Rotting flesh | Rotting flesh |
Cadaverine | Rotting flesh | Rotting flesh |
Pyridine | Fishy | |
Indole | Faecal, flowery | Faeces, jasmine |
Skatole | Faecal | Faeces |
The number of specific odour molecules associated with different plants, animals and other substances which produce a basis for computing different 'olfactory images' is unknown. We do know that the average human nose can distinguish between ten thousand and forty thousand odours. Professional smellers (perfumers, whisky blenders, wine judges and chefs) may be able to discriminate upward of one hundred thousand odours. The difference between an expert and an average nose is training.(1) But even the best noses don't make us the superior species in this respect.
For most domestic and wild animals a much larger proportion of their physiology is directly or indirectly concerned with smell. Yet, of the thousand or so genes dedicated to odour receptors in humans, there are a surprising number of pseudogenes (DNA sequences that are remnants of genes that are no longer functional). Compared to the number of olfactory pseudogenes in dogs (around 15%), for example, the number in humans is around 65% so only about 3-400 olfactory receptor genes in humans are actually functional. The upshot is that a disproportionate percentage of genes were at some stage committed to olfaction (1 percent) - far more genetic devotion than anything else in our bodies and brains get (for vision there are only four genes).
Attempting to account for this, one current theory posits that as mammals evolved there was an exchange of importance between smell and sight : "The better you can see, the less acutely you need to smell". The brains capacity for one or the other being finite led to superior visual detection winning out over smell.
Many animals continue to depend much more immediately on smell than we do. Their peculiar physiology combined with shorter life spans are more pressingly governed by the need to move towards nutrients, avoid poisons, seek out mates via the chemical attractants they release, determine territorial boundaries, detect predators as well as provide a means of communicating fear and anxiety. The drive that tells their motion systems what to do is essentially olfactory.
The sensitivity of many animal and insect species is nothing short of astonishing. Olfactory receptors which are near to theoretical limits respond to the absorption of just one or two molecules, as is the case with silk moths, which like most insects primarily smell via antennae. Salmon utilize their sense of smell to identify and return to their home stream waters. Smell is absolutely essential for a newborn mammalian pup to find the teats of its mother and obtain milk . Dogs, which can smell one to ten million times more acutely than humans, (depending on the breed) are considered to use their noses like humans do sight "...they have an olfactory geography, operating within a space whose points and boundaries are determined by smell."(2) Bloodhounds can pick up a day old trail and successfully identify its creator. The Silvertip Grizzly bear has a sense of smell seven times stronger than the Bloodhound, and is capable of smelling food from up to 18 miles away. Anecdotal evidence suggests even the smell of fear is discernible to animals, and by a few unlucky humans who have been placed in life threatening situations, sensing an odour reminiscent of decaying flesh.
Humans, monkeys and some aquatic species have a less developed sense of smell than other animals, as seen from a comparative diagram of the sizes of various olfactory bulbs.
Species differences. The area of the olfactory epithelium (red) in dogs is some forty times larger than in humans. Mice have about one thousand different odorant receptor types. Humans have a smaller number than mice; some of the genes have been lost during evolution. There are several millions of olfactory receptor cells in our olfactory epithelium.
Source: http://www.nobelprize.org/nobel_prizes/medicine/laureates/2004/illpres/illpres.html
Despite the differences, humans and animals seem to experience a similar spectrum of aromas, only we require them at greater concentrations. A dog can detect odours that simply don't exist for us because it's sensitive to concentrations nearly 100 million times lower.
But olfaction has turned out to be much more than 'just smelling.'
It is an enigmatic mind-body interface, influencing mood, memory, emotions, mate choice, the immune system and the endocrine (hormonal) system. Illustrated below are the main structures in the human olfactory system. With a glass of wine close to our noses, sniffing, we can imagine hitching a ride with the aroma molecules. We're traversing a complicated route through the olfactory apparatus that scientists have only recently begun to map.
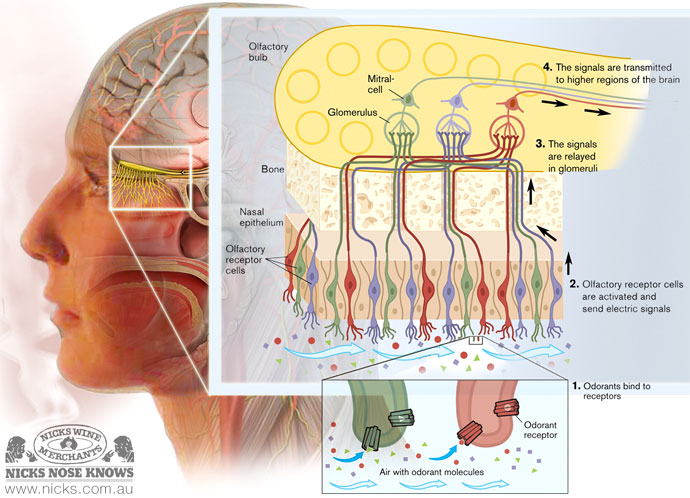
The sequence of events that occurs when we smell is summarised in the diagram above:
1. Odorant molecules enter via the nose and/or mouth via the retronasal passage.
2. The first level of odour detection takes place when the odorants bind to receptor cells in the upper part of the olfactory epithelium, which then send electrical signals up to the olfactory bulb.
3. In the olfactory bulb, these signals are relayed to glomeruli, collections of receptor cells of the same type that create a 'odour pattern' or profile.
4. The glomeruli receptor nerve endings excite mitral cells that forward the signal onto higher brain regions with maintained specificity.
5. The intensity of a given odour is determined by the Amygdala, the part of the brain responsible for emotion.
6. The character of a given odour is determined in the pre-frontal lobe.
7. Mucus helps to flush out odours to keep the sense of olfaction fresh and re-start the process.
Summary adapted from http://www.nobelprize.org/nobel_prizes/medicine/laureates/2004/illpres/illpres.html
Gordon Shepherd, a neuro scientist at Yale University once observed,"We think we smell with our noses [but] this is a little like saying that we hear with our ear lobes". In fact, the part of the nose we can see from the outside serves only to channel, filter, warm and humidify the air containing the chemicals of fragrance we call odorants.
The first of these receptors lie buried inside the brain, just below the eyes at the very top of the nasal cavity in a thumbnail size patch of cells called the nasal epithelium. This mucus-coated region at the roof of each nostril is typically 5cm2 in size for humans, with about 20 million olfactory receptors covering the epithelium of our right and left nostrils, but larger for more scent-savvy animals (some forty times larger in dogs).
Millions of specialised olfactory receptor neurons have a sole function of detecting odour molecules. The part of the neuron that resides within the olfactory epithelium is called the dendric knob. From this extends a series of hair-like filaments, called cilia, which reach into the layer of mucus coating the surface of the epithelium. Scent molecules in the inner nasal chamber are dissolved in the mucus and bound to specialised proteins which subsequently assist in transporting the molecules through the mucus to the scent receptors which then send electrical signals up to the olfactory bulb.
In the olfactory bulb - the part that transmits smell information from the nose to the brain - signals are then relayed to glomeruli, collections of receptor cells of the same type. This 'circuit layout' in the olfactory bulb is thought to be responsible, in various degrees, for discrimination and sensitivity of odour detection as well as filtering out background odours to enhance the transmission of a few select odours. But it remains unclear which, if any, of these functions are performed exclusively by the olfactory bulb.
At present, there are a number of competing theories regarding the actual mechanism of odour coding and perception. It's been proposed that odour receptor nerve cells function like a key-lock system: If the airborne molecules of a certain chemical can fit into the lock, the nerve cell will respond. An alternative theory, the 'Vibration theory' proposed by Luca Turin(3), posits that odour receptors detect the frequencies of vibrations of odour molecules in the infrared range by 'electron tunneling'. There's still no single theory that explains the mechanisms of olfactory perception completely and satisfactorily.
Scientists have made some dramatic advances, especially since 1991. It was the year a paper was published that opened the flood gates of new research into olfaction and the rest of our senses. 'A Molecular Basis for Odor Recognition' was the work of two researchers at Howard Hughes Medical Institute, Linda Buck and Richard Axel and represented a triumph for molecular biology. In 2004 the pair were awarded the Nobel Prize for their research and ensuing discoveries regarding odorant receptors and the organization of the olfactory system.
![]() |
![]() |
Buck & Axel found that each olfactory neuron expresses only one or, at most, a small number of different receptor genes. That is, a particular neuron is specialised at detecting one type of odour molecule. Most odours are composed of multiple odorant molecules, and each odorant molecule activates several odorant receptors. This leads to a combinatorial code forming an 'odorant pattern' - somewhat like the colours in a patchwork quilt or in a mosaic. Buck & Axel suggest that"The brain is essentially saying something like. 'I am seeing activity in positions 1, 15 and 54 of the olfactory bulb, which correspond to odorant receptors, 1, 15 and 54, so that must be jasmine."
Combinatorial receptor codes: The odorant receptor family is used in a combinatorial manner to detect odorants and encode their unique identities. Different odorants are detected by different combinations of receptors and thus have different receptor codes. These codes are translated by the brain into diverse odour perceptions. The immense number of potential receptor combinations is the basis for our ability to distinguish and form memories of more than 10,000 different odorants.
Source: http://www.nobelprize.org/nobel_prizes/medicine/laureates/2004/illpres/illpres.html
In a sense, the receptors break the odorant down and then the brain puts the odorant back together for identification. A kind of 'chemotopic' map in the brain shows specific activation patterns for specific odorants. This may be the basis for our ability to recognize and form memories of so many different odours.
Once registered and processed in the olfactory bulb, the odour information is relayed to some curious areas of the brain. So primal is our sense of smell that it bypasses the thalamus, which is typically responsible for sending information into the cortex. Instead, the sensation of a scent goes straight from the olfactory bulb to the cortex. Here, in the brain's executive region, namely the prefrontal cortex, a smell is determined to be either pleasant or unpleasant. A second brain region, the amygdala, perceives a smell's intensity. Interestingly, the primary function of amygdala is in the processing of memory and emotions, which helps to explain why a particular smell will trigger an emotional response toward an event from the past, and why the evaluation of a scent can often be so subjective.
Back in the lower chambers of the nose, the layer of mucus continues to moves at a rate of 10 to 60 mm per minute toward the nasopharynx (the part that connects the nasal cavity downwards to the pharynx). Its movement assists in the removal of odours after they've been sensed and so the cycle of smell begins anew.
It should be noted that, because the nose shares an airway, the pharynx, with the mouth, we smell and taste food simultaneously. "A flavour is simply an aroma you can eat", so when we speak of flavour we're referring to the summation of smell and taste. Or as the 18th century French gastronome, Brillat-Savarin put it, rather more quaintly: "...smell and taste form a single sense, of which the mouth is the laboratory and the nose is the chimney; or, to speak more exactly, of which one serves for the tasting of actual bodies and the other for the savoring of their gases".(4)
There are two ways this works. "As you eat, you smell your food twice: Once through your nose as food approaches it on the way to your mouth, called orthonasal olfaction, and again when the food is in your mouth, called retronasal olfaction, when the odors emitted from foods and beverages move from the mouth, back (thus "retro") and up behind the roof of the mouth into the nasal cavity. Retronasally perceived odors seem to come from "taste" in our mouth, but this is an illusion. The fact that people feel sure they have lost their sense of taste when they lose their sense of smell demonstrates how strong this illusion is. Our brains trick us by seamlessly knitting together the sensations of taste and smell to give us the flavours that make up our food world."(5)
70% to 75% of what we 'taste' (i.e.-flavour) is actually due to our olfactory system. If we are so unlucky to have caught a bad cold or sinus infection our nasal passages can become clogged with mucus, thus blocking the passage of air and odour molecules to the receptor cells. You will still be able to distinguish salty from sweet or bitter from sour due to the function of the tongue, but the finer details of 'flavour' are lost without the benefits of olfaction.
In this dramatically simplified overview our scope has been necessarily limited. The science of smell is extraordinarily complex and the breadth of the subject enormous. For the laymen, the journey begins realising that we occupy a culture of smell censorship where it's difficult to remember that olfaction is an instrument of knowledge. Smells can lead to a world of information and imagery if we let them. We encourage you to develop your own dictionary of the scents that waft into your world and you might discover a new vision beyond the autocracy of your other senses.
This chapter began by defining an odour to be a particular kind of molecule, with specific properties. We also gave some examples of odorous compounds and their typical 'smell'. We then looked into the biology of the sense of smell from a human perspective, detailing the structure of the olfactory system. In the next sections, we'll relate this back to our experience of wine, examining it from an olfactory perspective, discussing how particular aromas come about from various stages in the winemaking process and which aromas are considered faults and flaws in a wine. We'll conclude by considering how you can maximise your olfactory experience with wine.
Footnotes
* This is the case with methoxypyrazine, for example, the odour that is responsible for the 'green leaf', 'vegetative' smell in Sauvignon Blanc wines which sometimes intrudes into Cabernets.
Bibliography
1. The Scent of Desire, Rachel Herz. Harper Perenninal Publishing 2008
2. Appreciating Whisky, Phillip Hills. Harper Collins 2002
3. The Secret of Scent: Adventures in Perfume and the Science of Smell. Luca Turin, New York, 2006
4. On Food & Cooking. The Science and Lore of the Kitchen. Harold McGee. Unwin Hyman. 1984
5. The Scent of Desire, Rachel Herz. Harper Perenninal Publishing 2008